Stars exist as gigantic nuclear reactors: in the interior of a star, the fusion of light elements (e.g., hydrogen) into heavier elements (e.g., helium) yields a continuous supply of energy, and that energy ultimately balances the inward pull of gravity. Eventually, however, the star exhausts its supply of nuclear fuel, because the fusion of elements heavier than iron does not produce excess energy. Once the star reaches the point in its life where its core (the inner regions of the star that are densest) is composed primarily of iron, it has no choice but to collapse in on itself in an implosion, resulting in the formation of a neutron star. Remarkably, many massive stars that experience this implosion find a way to reverse the implosion into an extraordinarily luminous explosion, and these explosions — known as core-collapse supernovae (CCSne) — outshine the entire galaxy in which the dying star — and the newly formed neutron star — resides.
At Syracuse University we study various aspects of CCSne, one of which uses gravitational waves: as the core of the dying star implodes and subsequently explodes, time-dependent asymmetries in the distribution of mass in the core and in the shock — which is the surface/membrane that separates the rebounding and exploding core from the surrounding and infalling envelope — produce gravitational waves. We study the physics of the collapse and the properties of the shock analytically and with simplified models, to understand how instabilities operate and produce convection that can seed the gravitational-wave production. We also study the detectability of gravitational waves from CCSne and their spectra, i.e., the frequencies of the gravitational waves produced by CCSne and where (in frequency) most of the energy in gravitational waves resides (see Figure), and seek to relate the gravitational-wave energy and spectra to physical properties of the natal neutron star.
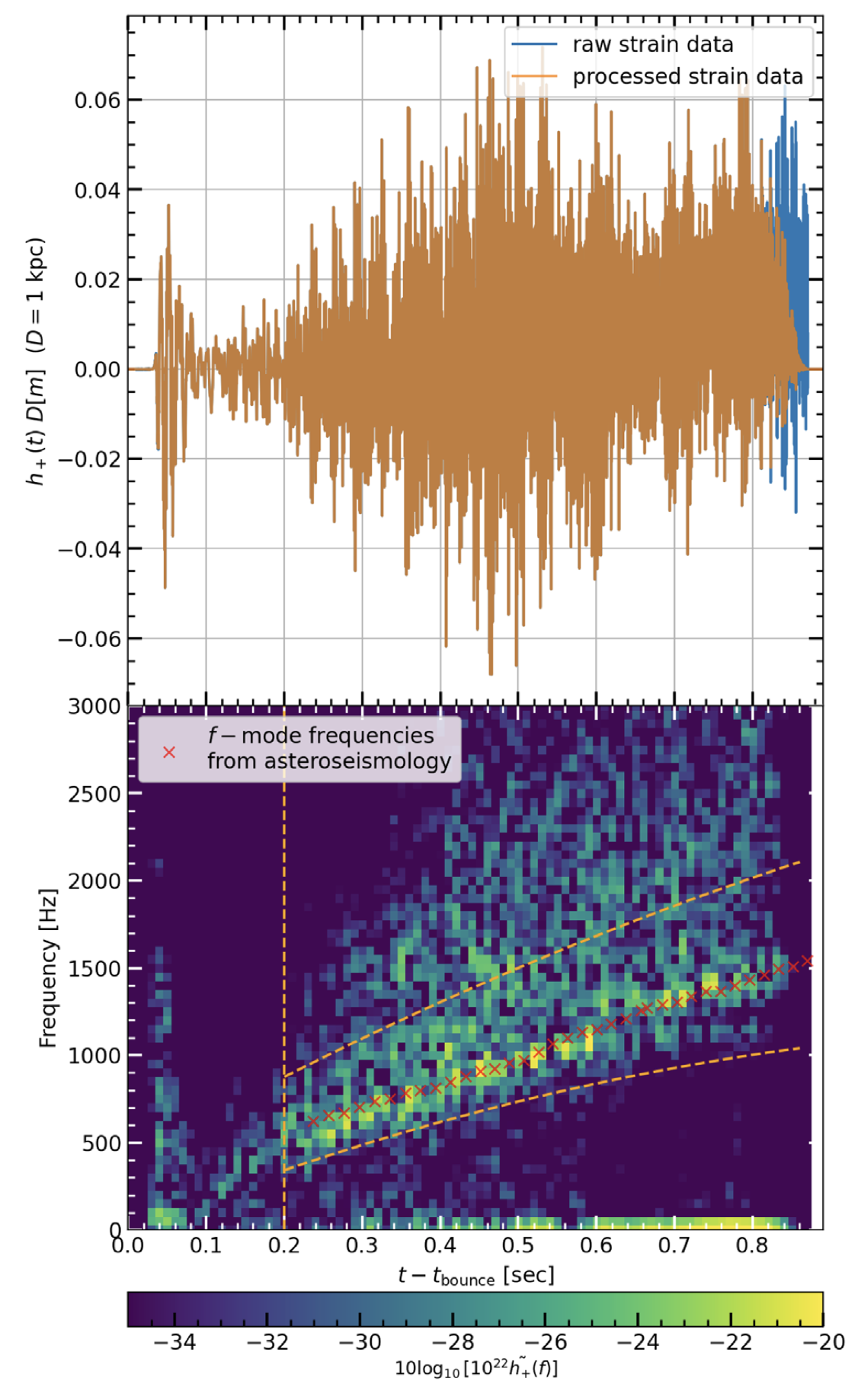
We also analyze weak and failed explosions: in come cases the dying star may not be able produce a successful CCSn, and instead continues to implode to the point of black hole formation. These “failed supernovae,” however, can be accompanied by a relatively weak (but still detectable) explosion as a consequence of the neutron-star formation and the liberation of neutrinos — elementary particles that accompany the de-leptonization of the collapsing core. We investigate the production and propagation of a second shock that is a byproduct of the neutrino emission (see Figure), as well as the accretion onto the black hole at the center of the dying star, ultimately to make connections to observations of extreme astrophysical transients.
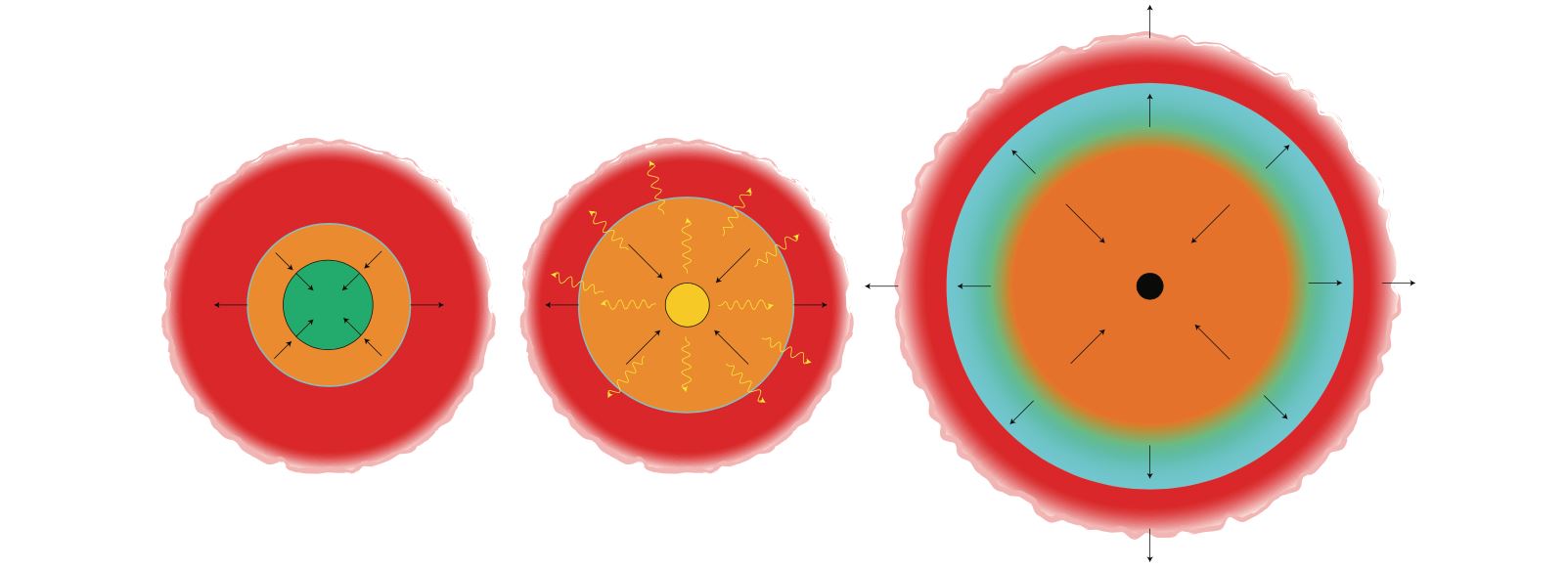